Aspirator
23.58
Diposting oleh Melany Christy

Operation
The cheap and simple water aspirator is the most common type of aspirator. It is used in chemistry and biology laboratories and consists of a tee fitting which is attached to a faucet and has a hose barb at one side. The flow of water passes through the straight portion of the tee, which has a restriction at the intersection, where the hose barb is attached. The vacuum hose should be connected to this barb.
If a liquid is used as the working fluid, the strength of the vacuum produced is limited by the vapor pressure of the liquid (for water, 3.2 kPa (32 mbar) at 25 degrees Celsius.) If a gas is used, however, this restriction does not exist. The industrial steam ejector (also called the steam jet ejector, steam aspirator, or steam jet aspirator) uses steam as a working fluid.
In order to avoid using too much steam, a single steam ejector stage is generally not used to generate vacuum below approximately 10 kPa (75 mmHg).[1] To generate higher vacuum, multiple stages are used; in a two-stage steam ejector, for example, the second stage provides vacuum for the waste steam output by the first stage. Condensers may be used between stages to reduce the load on the later stages. Steam ejectors with two, three, four, five and six stages may be used to produce vacuums down to 2.5 kPa, 300 Pa, 40 Pa, 4 Pa, and 0.4 Pa, respectively.[1]
The air ejector or venturi pump is similar to the steam ejector but uses high-pressure air as the working fluid. Multistage air ejectors can be used, but since air cannot easily be condensed at room temperature, an air ejector is usually limited to two or three stages.
Beryllium
23.53
Diposting oleh Melany Christy
Beryllium is the chemical element with the symbol Be and atomic number 4.
A bivalent element, beryllium is found naturally only combined with other elements in minerals. Notable gemstones which contain beryllium include beryl (aquamarine, emerald) and chrysoberyl. The free element is a steel-gray, strong, lightweight brittle alkaline earth metal. It is primarily used as a hardening agent in alloys, notably beryllium copper. Structurally, beryllium's very low density (1.85 times that of water), high melting point (1278 °C), high temperature stability, and low coefficient of thermal expansion, make it in many ways an ideal aerospace material, and it has been used in rocket nozzles and is a significant component of planned space telescopes. Because of its relatively high transparency to X-rays and other ionizing radiation types, beryllium also has a number of uses as filters and windows for radiation and particle physics experiments.
Commercial use of beryllium metal presents technical challenges due to the toxicity (especially by inhalation) of beryllium-containing dusts. Beryllium produces a direct corrosive effect to tissue, and can cause a chronic life-threatening allergic disease called berylliosis in susceptible persons.
Beryllium is a relatively rare element in both the Earth and the universe. The element is not known to be necessary or useful for either plant or animal life.
Appearance | ||||||||||||||||||||||||||||
---|---|---|---|---|---|---|---|---|---|---|---|---|---|---|---|---|---|---|---|---|---|---|---|---|---|---|---|---|
white-gray metallic![]() | ||||||||||||||||||||||||||||
General properties | ||||||||||||||||||||||||||||
Name, symbol, number | beryllium, Be, 4 | |||||||||||||||||||||||||||
Pronunciation | /bəˈrɪliəm/, bə-RIL-ee-əm | |||||||||||||||||||||||||||
Element category | alkaline earth metal | |||||||||||||||||||||||||||
Group, period, block | 2, 2, s | |||||||||||||||||||||||||||
Standard atomic weight | 9.012182(3) g·mol−1 | |||||||||||||||||||||||||||
Electron configuration | 1s2 2s2 | |||||||||||||||||||||||||||
Electrons per shell | 2, 2 (Image) | |||||||||||||||||||||||||||
Physical properties | ||||||||||||||||||||||||||||
Phase | solid | |||||||||||||||||||||||||||
Density (near r.t.) | 1.85 g·cm−3 | |||||||||||||||||||||||||||
Liquid density at m.p. | 1.690 g·cm−3 | |||||||||||||||||||||||||||
Melting point | 1560 K, 1287 °C, 2349 °F | |||||||||||||||||||||||||||
Boiling point | 2742 K, 2469 °C, 4476 °F | |||||||||||||||||||||||||||
Heat of fusion | 12.2 kJ·mol−1 | |||||||||||||||||||||||||||
Heat of vaporization | 297 kJ·mol−1 | |||||||||||||||||||||||||||
Specific heat capacity | (25 °C) 16.443 J·mol−1·K−1 | |||||||||||||||||||||||||||
Vapor pressure | ||||||||||||||||||||||||||||
| ||||||||||||||||||||||||||||
Atomic properties | ||||||||||||||||||||||||||||
Oxidation states | 2, 1[1] (amphoteric oxide) | |||||||||||||||||||||||||||
Electronegativity | 1.57 (Pauling scale) | |||||||||||||||||||||||||||
Ionization energies (more) | 1st: 899.5 kJ·mol−1 | |||||||||||||||||||||||||||
2nd: 1757.1 kJ·mol−1 | ||||||||||||||||||||||||||||
3rd: 14848.7 kJ·mol−1 | ||||||||||||||||||||||||||||
Atomic radius | 105[2] pm | |||||||||||||||||||||||||||
Atomic radius (calc.) | 112 [3] pm | |||||||||||||||||||||||||||
Covalent radius | 96±3 pm | |||||||||||||||||||||||||||
Van der Waals radius | 153 pm | |||||||||||||||||||||||||||
Miscellanea | ||||||||||||||||||||||||||||
Crystal structure | hexagonal | |||||||||||||||||||||||||||
Magnetic ordering | diamagnetic | |||||||||||||||||||||||||||
Electrical resistivity | (20 °C) 36 nΩ·m | |||||||||||||||||||||||||||
Thermal conductivity | (300 K) 200 W·m−1·K−1 | |||||||||||||||||||||||||||
Thermal expansion | (25 °C) 11.3 µm·m−1·K−1 | |||||||||||||||||||||||||||
Speed of sound (thin rod) | (r.t.) 12870[4] m·s−1 | |||||||||||||||||||||||||||
Young's modulus | 287 GPa | |||||||||||||||||||||||||||
Shear modulus | 132 GPa | |||||||||||||||||||||||||||
Bulk modulus | 130 GPa | |||||||||||||||||||||||||||
Poisson ratio | 0.032 | |||||||||||||||||||||||||||
Mohs hardness | 5.5 | |||||||||||||||||||||||||||
Vickers hardness | 1670 MPa | |||||||||||||||||||||||||||
Brinell hardness | 600 MPa | |||||||||||||||||||||||||||
CAS registry number | 7440-41-7 | |||||||||||||||||||||||||||
Most stable isotopes | ||||||||||||||||||||||||||||
Main article: Isotopes of beryllium | ||||||||||||||||||||||||||||
|
History
![]() | This section requires expansion. |
Beryllium was discovered by Louis-Nicolas Vauquelin in 1798 as a component of beryl and in emeralds. Friedrich Wöhler[5] and Antoine Bussy independently isolated the metal in 1828 by reacting potassium and beryllium chloride. Beryllium's chemical similarity to aluminum was probably why beryllium was missed in previous searches.[6]
[edit] Etymology
The name beryllium comes (via Latin: Beryllus and French: Béryl) from the Greek βήρυλλος, bērullos, beryl, from Prakrit veruliya (वॆरुलिय), from Pāli veḷuriya (वेलुरिय); veḷiru (भेलिरु) or, viḷar (भिलर्), "to become pale," in reference to the pale semiprecious gemstone beryl.[7] The original source of the word "Beryllium" is the Sanskrit word: वैडूर्य vaidurya-, which is of Dravidian origin and could be derived from the name of the modern city of Belur.[8] For about 160 years, beryllium was also known as glucinum or glucinium (with the accompanying chemical symbol "Gl"[9]), the name coming from the Greek word for sweet: γλυκυς, due to the sweet taste of its salts.
Characteristics
Physical
Beryllium has one of the highest melting points of the light metals. It has exceptional elastic rigidity (Young's modulus 287 GPa). The modulus of elasticity of beryllium is approximately 50% greater than that of steel. The combination of this modulus plus beryllium's relatively low density gives it an unusually fast sound conduction speed at standard conditions (about 12.9 km/s). Other significant properties are the high values for specific heat (1925 J·kg−1·K−1) and thermal conductivity (216 W·m−1·K−1), which make beryllium the metal with the best heat dissipation characteristics per unit weight. In combination with the relatively low coefficient of linear thermal expansion (11.4 × 10−6 K−1), these characteristics ensure that beryllium demonstrates a unique degree of dimensional stability under conditions of thermal loading.[10]
At standard temperature and pressures beryllium resists oxidation when exposed to air (its ability to scratch glass is due to the formation of a thin layer of the hard oxide BeO). It resists corrosion by concentrated nitric acid.[11]
Nuclear
Beryllium has a large scattering cross section for high-energy neutrons, thus effectively slowing the neutrons to the thermal energy range where the cross section is low (0.008 barn). The predominant beryllium isotope 9Be also undergoes a (n,2n) neutron reaction to 8Be, that is beryllium is a neutron multiplier, releasing more neutrons than it absorbs. Beryllium is highly permeable to X-rays, and neutrons are liberated when it is hit by alpha particles.[10]
Isotopes


Of beryllium's isotopes, only 9Be is stable and the others are relatively unstable or rare. It is thus a mononuclidic element.
Cosmogenic 10Be is produced in the atmosphere by cosmic ray spallation of oxygen and nitrogen. Cosmogenic 10Be accumulates at the soil surface, where its relatively long half-life (1.36 million years) permits a long residence time before decaying to 10B. Thus, 10Be and its daughter products have been used to examine soil erosion, soil formation from regolith, the development of lateritic soils, as well as variations in solar activity and the age of ice cores. Solar activity is inversely correlated with 10Be production, because solar wind decreases flux of galactic cosmic rays which reach Earth.
Nuclear explosions also form 10Be by a reaction of fast neutrons with 13C in the carbon dioxide in air. This is one of the historical indicators of past activity at nuclear test sites.[12]
The fact that 7Be and 8Be have very short half-lives has profound cosmological consequences. Elements heavier than beryllium could not be produced by nuclear fusion in the Big Bang. This is due to the lack of sufficient time during the Big Bang nucleosynthesis phase to produce carbon by fusion of 4He nuclei and the very low concentrations of available 8Be. Astronomer Fred Hoyle first showed that the energy levels of 8Be and 12C allow carbon production by the triple-alpha process in helium-fueled stars where more synthetic time is available, thus making life possible from the supernova "ash" from these stars. (See also Big Bang nucleosynthesis).[13]
7Be decays by electron capture, therefore its decay rate is dependent upon its electron configuration – a rare occurrence in nuclear decay.[14]
The shortest-lived known isotope of beryllium is 13Be which decays through neutron emission. It has a half-life of 2.7 × 10−21 second. 6Be is also very short-lived with a half-life of 5.0 × 10−21 second.[11]
The exotic isotopes 11Be and 14Be are known to exhibit a nuclear halo.[15]
Chemical
Beryllium has the electronic configuration [He] 2s2. In its chemistry beryllium exhibits the +2 oxidation state and the only evidence of lower valence of beryllium is in the solubility of the metal in BeCl2.[16] The small atomic radius ensures that the Be2+ ion would be highly polarizing leading to significant covalent character in beryllium's bonding.[17] Beryllium is 4 coordinate in complexes e.g. [Be(H2O)4]2+ and tetrahaloberyllates, BeX2−4. This characteristic is used in analytical techniques using EDTA as a ligand which preferentially forms octahedral complexes – thus absorbing other cations such as Al3+ which might interfere, for example in the solvent extraction of a complex formed between Be2+ and acetylacetone.[18]
Beryllium metal sits above aluminium in the electrochemical series and would be expected to be a reactive metal, however it is passivated by an oxide layer and does not react with air or water even at red heat.[17] Once ignited however beryllium burns brilliantly forming a mixture of beryllium oxide and beryllium nitride.[17] Beryllium dissolves readily in non-oxidizing acids, such as HCl and H2SO4, but not in nitric as this forms the oxide and this behavior is similar to that of aluminium metal. Beryllium, again similarly to aluminium, dissolves in warm alkali to form the beryllate anion, Be(OH)2−4, and hydrogen gas. The solutions of salts, e.g. beryllium sulfate and beryllium nitrate are acidic because of hydrolysis of the [Be(H2O)4]2+ ion; for example
- [Be(H2O)4]2+ + H2O
[Be(H2O)3(OH)]+ + H3O+
Compounds
Beryllium forms binary compounds with many non-metals. Beryllium hydride is an amorphous white solid believed to be built from corner-sharing {BeH4} tetrahedra.[19]
All four anhydrous halides are known. BeF2 has a silica-like structure with corner-shared BeF4 tetrahedra. BeCl2 and BeBr2 have chain structures with edge-shared tetrahedra. They all have linear monomeric gas phase forms.[17]
Beryllium oxide, BeO, is a white, high-melting-point solid, which has the wurtzite structure with a thermal conductivity as high as some metals. BeO is amphoteric. Beryllium hydroxide, Be(OH)2 has low solubility in water and is amphoteric. Salts of beryllium can be produced by reacting Be(OH)2 with acid.[17]
Beryllium sulfide, selenide and telluride all have the zincblende structure.[16]
Beryllium nitride, Be3N2 is a high-melting-point compound which is readily hydrolyzed. Beryllium azide, BeN6 is known and beryllium phosphide, Be3P2 has a similar structure to Be3N2.[16]
A number of beryllium borides are known, Be5B, Be4B, Be2B, BeB2, BeB6, BeB12.[16]
Beryllium carbide, Be2C, is a high melting, brick red compound that reacts with water to give methane.[16] No beryllium silicide has been identified.[17]
Basic beryllium nitrate and basic beryllium acetate have similar tetrahedral structures with four beryllium atoms coordinated to a central oxide ion.[16]
Occurrence
The beryllium concentration of the Earth's surface rocks is ca. 4–6 ppm. Beryllium is a constituent of about 100 out of about 4000 known minerals, the most important of which are bertrandite (Be4Si2O7(OH)2), beryl (Al2Be3Si6O18), chrysoberyl (Al2BeO4), and phenakite (Be2SiO4). Precious forms of beryl are aquamarine, bixbite and emerald.[10]
Production
Because of its high affinity for oxygen at elevated temperatures and its ability to reduce water when its oxide film is removed, the extraction of beryllium from its compounds is very difficult. Although electrolysis of a fused mixture of beryllium and sodium fluorides was used to isolate the element in the nineteenth century, the metal's high melting point makes this process more energy intensive than the corresponding production of alkali metals. Early in the twentieth century, the production of beryllium by the thermal decomposition of beryllium iodide was investigated following the success of a similar process for the production of zirconium, but this proved to be uneconomic for volume production.[20]
Beryllium metal did not become readily available until 1957. Currently, most is produced by reducing beryllium fluoride with magnesium metal. The price on the US market for vacuum-cast beryllium ingots was 338 US$ per pound ($745/kg) in 2001.[21]
- BeF2 + Mg → MgF2 + Be
Applications
Radiation windows




Because of its low atomic number and very low absorption for X-rays, the oldest and still one of the most important applications of beryllium is in radiation windows for X-ray tubes. Extreme demands are placed on purity and cleanliness of Be to avoid artifacts in the X-ray images. Thin beryllium foils are used as radiation windows for X-ray detectors, and the extremely low absorption minimizes the heating effects caused by high intensity, low energy X-rays typical of synchrotron radiation. Vacuum-tight windows and beam-tubes for radiation experiments on synchrotrons are manufactured exclusively from beryllium. In scientific setups for various X-ray emission studies (e.g., energy-dispersive X-ray spectroscopy) the sample holder is usually made of beryllium because its emitted X-rays have much lower energies (~100 eV) than X-rays from most studied materials.[10]
Because of its low atomic number beryllium is almost transparent to energetic particles. Therefore it is used to build the beam pipe around the collision region in collider particle physics experiments. Notably all four main detector experiments at the Large Hadron Collider accelerator (ALICE, ATLAS, CMS, LHCb) use a beryllium beam-pipe.
Also many high-energy particle physics collision experiments such as the Large Hadron Collider, the Tevatron, the SLAC and others contain beam pipes made of beryllium. Beryllium's low density allows collision products to reach the surrounding detectors without significant interaction, its stiffness allows a powerful vacuum to be produced within the pipe to minimize interaction with gases, its thermal stability allows it to function correctly at temperatures of only a few degrees above absolute zero, and its diamagnetic nature keeps it from interfering with the complex multipole magnet systems used to steer and focus the particle beams.[22]
Mechanical
Because of its stiffness, light weight, and dimensional stability over a wide temperature range, beryllium metal is used for lightweight structural components in the defense and aerospace industries in high-speed aircraft, missiles, space vehicles and communication satellites. Several liquid-fuel rockets use nozzles of pure beryllium.[23][24]
Beryllium is used as an alloying agent in the production of beryllium copper, which contains up to 2.5% beryllium. Beryllium-copper alloys are used in many applications because of their combination of high electrical and thermal conductivity, high strength and hardness, nonmagnetic properties, along with good corrosion and fatigue resistance. These applications include the making of spot welding electrodes, springs, non-sparking tools and electrical contacts.
Beryllium was also used in Jason pistols which were used to strip paint from the hulls of ships. In this case, beryllium was alloyed to copper and used as a hardening agent.[25]
The excellent elastic rigidity of beryllium has led to its extensive use in precision instrumentation, e.g. in gyroscope inertial guidance systems, and in support structures for optical systems.[10]
Beryllium mirrors are a field of particular interest. Large-area mirrors, frequently with a honeycomb support structure, are used, for example, in meteorological satellites where low weight and long-term dimensional stability are critical. Smaller beryllium mirrors are used in optical guidance systems and in fire-control systems, e.g. in the German Leopard 1 and Leopard 2 main battle tanks. In these systems, very rapid movement of the mirror is required which again dictates low mass and high rigidity. Usually the beryllium mirror is coated with hard electroless nickel plating which can be more easily polished to a finer optical finish than beryllium. In some applications, though, the beryllium blank is polished without any coating. This is particularly applicable to cryogenic operation where thermal expansion mismatch can cause the coating to buckle.[10]
The James Webb Space Telescope[26] will have 18 hexagonal beryllium sections for its mirrors. Because JWST will face a temperature of 33 degrees K, the mirror is made of beryllium, capable of handling extreme cold better than glass. Beryllium contracts and deforms less than glass—and remains more uniform—in such temperatures.[27] For the same reason, the optics of the Spitzer Space Telescope are entirely built of beryllium metal.[28]
An earlier major application of beryllium was in brakes for military aircraft because of its hardness, high melting point and exceptional heat dissipation. Environmental considerations have led to substitution by other materials.[10]
Cross-rolled beryllium sheet is an excellent structural support for printed circuit boards in surface-mount technology. In critical electronic applications, beryllium is both a structural support and heat sink. The application also requires a coefficient of thermal expansion that is well matched to the alumina and polyimide-glass substrates. The beryllium-beryllium oxide composite "E-Materials" have been specially designed for these electronic applications and have the additional advantage that the thermal expansion coefficient can be tailored to match diverse substrate materials.[10]
Magnetic
Beryllium is non-magnetic. Therefore, beryllium-based tools are used by military naval EOD-personnel when working on or around naval mines, as these often have fuzes that detonate on direct magnetic contact or when influenced by a magnetic field.[29] They are also found in maintenance and construction materials near MRI scanners. Magnetic tools would be pulled by the scanner's strong magnetic field. Apart from being difficult to remove once magnetic items are stuck in the scanner, the missile-effect can have dangerous consequences.[30] In the telecommunications industry, tools made of beryllium are used to tune the highly magnetic klystrons used for high power microwave applications.
Nuclear
Beryllium is used in nuclear weapon designs as the outer layer of the pit of the primary stage, surrounding the fissile material. It is a good pusher for implosion, and a very good neutron reflector, as in beryllium moderated reactors.[31]
Beryllium is sometimes used in neutron sources, in which the beryllium is mixed with an alpha emitter such as 210Po, 226Ra, 239Pu or 241Am. The "Urchin" neutron initiator in early nuclear weapons used beryllium-polonium combination.[31]
Beryllium is used in the Joint European Torus fusion research facility and will be used in ITER, to condition the plasma facing components.[32] Beryllium has also been proposed as a cladding material for nuclear fuel, due to its combination of mechanical, chemical, and nuclear properties.[10]
Acoustics
Beryllium's characteristics (low weight and high rigidity) make it useful as a material for high-frequency speaker drivers. Until recently, most beryllium tweeters used an alloy of beryllium and other metals due to beryllium's high cost and difficulty to form. These challenges, coupled with the high performance of beryllium, caused some manufacturers to falsely claim using pure beryllium.[33] Some high-end audio companies manufacture pure beryllium tweeters or speakers using these tweeters. Because beryllium is many times more expensive than titanium, hard to shape due to its brittleness, and toxic if mishandled, these tweeters are limited to high-end and public address applications.[34][35][36]
Electronic
Beryllium is a p-type dopant in III-V compound semiconductors. It is widely used in materials such as GaAs, AlGaAs, InGaAs, and InAlAs grown by molecular beam epitaxy (MBE).[37]
Beryllium oxide is useful for many applications that require the combined properties of an electrical insulator and an excellent heat conductor, with high strength and hardness, and a very high melting point. Beryllium oxide is frequently used as an insulator base plate in high-power transistors in RF transmitters for telecommunications. Beryllium oxide is also being studied for use in increasing the thermal conductivity of uranium dioxide nuclear fuel pellets.[38]
Beryllium compounds were used in fluorescent lighting tubes, but this use was discontinued because of berylliosis in the workers manufacturing the tubes.[39]
Toxicity


The toxicity of beryllium depends upon the duration, intensity and frequency of exposure (features of dose), as well as the form of beryllium and the route of exposure (i.e. inhalation, dermal, ingestion). According to the International Agency for Research on Cancer (IARC), beryllium and beryllium compounds are Category 1 carcinogens; they are carcinogenic to both animals and humans.[40] Chronic berylliosis is a pulmonary and systemic granulomatous disease caused by exposure to beryllium. Acute beryllium disease in the form of chemical pneumonitis was first reported in Europe in 1933 and in the United States in 1943. Cases of chronic berylliosis were first described in 1946 among workers in plants manufacturing fluorescent lamps in Massachusetts. Chronic berylliosis resembles sarcoidosis in many respects, and the differential diagnosis is often difficult. It occasionally killed early workers in nuclear weapons design, such as Herbert Anderson.[41]
Although the use of beryllium compounds in fluorescent lighting tubes was discontinued in 1949, potential for exposure to beryllium exists in the nuclear and aerospace industries and in the refining of beryllium metal and melting of beryllium-containing alloys, the manufacturing of electronic devices, and the handling of other beryllium-containing material.
Early researchers tasted beryllium and its various compounds for sweetness in order to verify its presence. Modern diagnostic equipment no longer necessitates this highly risky procedure and no attempt should be made to ingest this highly toxic substance. Beryllium and its compounds should be handled with great care and special precautions must be taken when carrying out any activity which could result in the release of beryllium dust (lung cancer is a possible result of prolonged exposure to beryllium laden dust).
This substance can be handled safely if certain procedures are followed. No attempt should be made to work with beryllium before familiarization with correct handling procedures.
A successful test for beryllium in air and on surfaces has been recently developed and published as an international voluntary consensus standard (ASTM D7202; www.astm.org). The procedure uses dilute ammonium bifluoride for dissolution and fluorescence detection with beryllium bound to sulfonated hydroxybenzoquinoline, allowing detection up to 100 times lower than the recommended limit for beryllium concentration in the workplace. Fluorescence increases with increasing beryllium concentration. The new procedure has been successfully tested on a variety of surfaces and is effective for the dissolution and ultratrace detection of refractory beryllium oxide and siliceous beryllium (ASTM D7458).[42]
Inhalation
Beryllium is harmful if inhaled and the effects depend on the duration, intensity, and frequency of exposure. If beryllium concentrations in air are high enough (greater than 100 µg/m3), an acute condition can result, called acute beryllium disease, which resembles pneumonia. Occupational and community air standards are effective in preventing most acute lung damage. Long-term beryllium exposure can increase the risk of developing lung cancer. The more common serious health problem from beryllium today is chronic beryllium disease (CBD), discussed below. It continues to occur in industries as diverse as metal recycling, dental laboratories, alloy manufacturing, nuclear weapons production and metal machine shops that work with alloys containing small amounts of beryllium. A 2008 report from the United States National Research Council said that worker exposure to beryllium should be kept "at the lowest feasible level," as the agency's research could not establish any safe level of exposure.[43]
Acute beryllium disease
Overexposure to beryllium can cause inflammation of the upper and/or lower respiratory tracts. The symptoms of acute beryllium disease are non-specific, resembling other inhalational injuries, viral infections, or pneumonia[44]. Upper respiratory findings include nasopharyngitis and tracheobronchitis. Symptoms may include irritation of the nares and pharynx, epistaxis, cough, and a metallic taste. Nasopharyngitis can progress to formation of nasal fissures, ulcerations, or perforation. Therapy is supportive and includes removal from further beryllium exposure. Acute beryllium pneumonitis produces severe cough (occasionally with blood-streaked sputum), chest pain or burning, and shortness of breath[44]. The patient is usually ill-appearing, and present with hypoxemia, cyanosis, tachycardia, and shallow rapid breathing. Systemic symptoms include fever (usually low-grade), malaise, and anorexia. The chest X-ray can reveal diffuse, bilateral alveolar infiltrates. There is no specific diagnostic test for acute beryllium disease. Biopsy of the lungs reveals a nonspecific granulomatous inflammation. Treatment is supportive, including oxygen supplementation as needed, and removal from further beryllium exposure. Corticosteroids are sometimes tried, but there are no good controlled studies reported. The signs and symptoms of acute beryllium pneumonitis usually resolve over several weeks to months, but it may be fatal, and about 15-20% of cases may progress to CBD[45].
Chronic beryllium disease (CBD)
The average rate of sensitization to beryllium ranges from 1-5%, depending upon exposure details[46][47]. Sensitization is not an illness, but some of these individuals, if inhaling sufficient quantities of beryllium dust in the micrometer-size range, may have an inflammatory reaction that principally targets the respiratory system and skin. This condition is called chronic beryllium disease (CBD), and can occur within a few months or many years (average 10 years in some series[48]) after exposure to higher-than-normal levels of beryllium (greater than 0.2 µg/m3). This disease causes fatigue, weakness, night sweats and can cause difficulty in breathing and a persistent dry cough. It can result in anorexia, weight loss, and may also lead to right-side heart enlargement and heart disease in advanced cases. Typically, CBD has an insidious onset and runs an indolent course. Some people who are sensitized to beryllium may not have symptoms, and just being sensitized is not a recognized health effect. CBD occurs when the body's immune system recognizes beryllium particles as foreign material and mounts an immune system attack against the particles. Because these particles are typically inhaled into the lungs, the lungs become the major site where the immune system responds. The lung sacs become inflamed and fill with large numbers of white blood cells that accumulate wherever beryllium particles are found. These cells form balls around the beryllium particles called "granulomas." When enough of these develop, they interfere with the normal function of the organ. Over time, the lungs become stiff and lose their ability to help transfer oxygen from the air into the bloodstream. Early on in the disease, there may be a mild obstructive ventilatory defect. In advanced cases, there is a restrictive defect and reduced diffusion capacity. Patients with CBD develop difficulty inhaling and exhaling sufficient amounts of air, and the amount of oxygen in their bloodstreams falls. Most of the clinical features of CBD are indistinguishable from thoracic involvement with sarcoidosis. The chest radiograph usually reveals bilateral, mid- and upper-lobe predominant reticulonodular infiltrates, as well as hilar and mediastinal adenopathy. For suspected cases of CBD, fiber-optic bronchoscopy with bronchoalveolar lavage (BAL) is indicated. The BAL fluid should undergo beryllium lymphocyte proliferation testing (BAL BeLPT), the "gold standard" for the diagnosis. The measurement of beryllium in urine or tissue does not establish the diagnosis, and there are technical and interpretive problems with these tests. Blood BeLPT detects approximately 70-90% of CBD cases. CBD is treatable, but not curable with traditional drugs and medicine. Removal from further beryllium exposure is advisable. Treatment includes supplemental oxygen and corticosteroids (such as prednisone) to lower the body's overreaction to beryllium. If therapy with corticosteroid is successful, treatment is usually continued lifelong because of disease relapse after steroid cessation[44]. In general, CBD worsens without treatment. Prognosis is best for those diagnosed at an earlier stage. Overall mortality rates are 5-38%[49]. The general population is unlikely to develop acute or chronic beryllium disease because ambient air levels of beryllium are normally very low (<0.03>3).[50]
Ingestion
Swallowing beryllium has not been reported to cause effects in humans because very little beryllium is absorbed from the stomach and intestines. Harmful effects have sometimes been seen in animals ingesting beryllium.[51]
Dermatological effects
Beryllium can cause local irritation and contact dermatitis. Beryllium contact with skin that has been scraped or cut may cause rashes, ulcers, or bumps under the skin called granulomas.[52] Beryllium dust or powder can irritate the eyes, producing itching, burning, or conjunctivitis.
Effects on children
There are no studies on the health effects of children exposed to beryllium, although individual cases of CBD have been reported in children of beryllium workers from the 1940s. It is unknown whether children differ from adults in their susceptibility to beryllium. It is unclear whether beryllium is teratogenic.[53]
Detection in the body
Beryllium can be measured in the urine and blood. The amount of beryllium in blood or urine may not indicate time or quantity of exposure. Beryllium levels can also be measured in lung and skin samples. While such measurements may help establish that exposure has occurred, other tests are used to determine if that exposure has resulted in health effects. A blood test, the blood beryllium lymphocyte proliferation test (BeLPT), identifies beryllium sensitization and has predictive value for CBD. The BeLPT has become the standard test for detecting beryllium sensitization and CBD in individuals who are suspected of having CBD and to help distinguish it from similar conditions such as sarcoidosis. It is also the main test used in industry health programs to monitor whether disease is occurring among current and former workers who have been exposed to beryllium on the job. The test can detect disease that is at an early stage, or can detect disease at more advanced stages of illness as well. The BeLPT can also be performed using cells obtained from a person's lung by a procedure called "bronchoscopy".[54]
Industrial release and occupational exposure limits
Typical levels of beryllium that industries may release into the air are of the order of 0.01 µg/m3, averaged over a 30-day period, or 2 µg/m3 of workroom air for an 8-hour work shift. Compliance with the current U.S. Occupational Safety and Health Administration (OSHA) permissible exposure limit for beryllium of 2 µg/m3 has been determined to be inadequate to protect workers from developing beryllium sensitization and CBD. The American Conference of Governmental Industrial Hygienists (ACGIH), which is an independent organization of experts in the field of occupational health, has proposed a threshold limit value (TLV) of 0.05 µg/m3 in a 2006 Notice of Intended Change (NIC). This TLV is 40 times lower than the current OSHA permissible exposure limit, reflecting the ACGIH analysis of best available peer-reviewed research data concerning how little airborne beryllium is required to cause sensitization and CBD. Because it can be difficult to control industrial exposures to beryllium, it is advisable to use any methods possible to reduce airborne and surface contamination by beryllium, to minimize the use of beryllium and beryllium-containing alloys whenever possible, and to educate people about the potential hazards if they are likely to encounter beryllium dust or fumes.[55]
On 29 January 2009, the Los Alamos National Laboratory announced it was notifying nearly 2,000 current and former employees and visitors that they may have been exposed to beryllium in the lab and may be at risk of disease. Concern over possible exposure to the material was first raised in November 2008, when a box containing beryllium was received at the laboratory's short-term storage facility.[56]
BBeaker (glassware)
23.53
Diposting oleh Melany Christy

Structure
Standard or "Low-form" beakers typically have a height about 1.4 times the diameter.[2] The common low form with a spout has been called the Griffin form.[3] "Tall form" beakers have a height about twice the diameter.[2] These are sometimes called Berzelius beakers.
A beaker is distinguished from a flask by having sides which are straight rather than sloping. The exception to this definition is a slightly conical sided beaker called a Phillips beaker.
Materials
Beakers are commonly made of glass (today usually borosilicate glass[2]), but can also be in metal (such as stainless steel or aluminium) or certain plastics, (notably polythene, polypropylene, PTFE). A common use for polypropylene beakers is gamma spectral analysis of liquid and solid samples.
Shape
Beakers are often graduated, that is, marked on the side with lines indicating the volume contained. For instance, a 250 mL beaker might be marked with lines to indicate 50, 100, 150, 200, and 250 mL of volume. These marks are not intended for obtaining a precise measurement of volume (a graduated cylinder would be a more appropriate instrument for such a task), but rather an estimation.
The presence of a lip means that the beaker cannot have a lid. However, when in use, beakers may be covered by a watch glass to prevent contamination or loss of the contents, but allowing venting via the spout.
Lithium
23.45
Diposting oleh Melany Christy
Lithium is a soft, silver-white metal that belongs to the alkali metal group of chemical elements. It is represented by the symbol Li, and it has the atomic number 3. Under standard conditions it is the lightest metal and the least dense solid element. Like all alkali metals, lithium is highly reactive, corroding quickly in moist air to form a black tarnish. For this reason, lithium metal is typically stored under the cover of petroleum. When cut open, lithium exhibits a metallic luster, but contact with oxygen quickly turns it back to a dull silvery gray color. Lithium in its elemental state is highly flammable.
According to one cosmogenic theory, lithium was one of the few elements synthesized in the Big Bang, albeit in relatively small quantities. Since its current estimated abundance in the universe is vastly less than that predicted by physical theories, the processes by which new lithium is created and destroyed, and the true value of its abundance,[1] continue to be active matters of study in astronomy.[2][3][4] The nuclei of lithium are relatively fragile: the two stable lithium isotopes found in nature have lower binding energies per nucleon than any other stable compound nuclides, save deuterium, and helium-3 (3He).[5] Though very light in atomic weight, lithium is less common in the solar system than 25 of the first 32 chemical elements.[6]
Because of its high reactivity, lithium only appears naturally in the form of compounds. Lithium occurs in a number of pegmatitic minerals, but is also commonly obtained from brines and clays. On a commercial scale, lithium metal is isolated electrolytically from a mixture of lithium chloride and potassium chloride.
Trace amounts of lithium are present in the oceans and in some organisms, though the element serves no apparent vital biological function in humans. The lithium ion Li+ administered as any of several lithium salts has proved to be useful as a mood stabilizing drug due to neurological effects of the ion in the human body. Lithium and its compounds have several industrial applications, including heat-resistant glass and ceramics, high strength-to-weight alloys used in aircraft, and lithium batteries. Lithium also has important links to nuclear physics. The transmutation of lithium atoms to tritium was the first man-made form of a nuclear fusion reaction, and lithium deuteride serves as a fusion fuel in staged thermonuclear weapons.
Appearance | |||||||||||||||||||||||||
---|---|---|---|---|---|---|---|---|---|---|---|---|---|---|---|---|---|---|---|---|---|---|---|---|---|
silvery white (seen here in oil)![]() | |||||||||||||||||||||||||
General properties | |||||||||||||||||||||||||
Name, symbol, number | lithium, Li, 3 | ||||||||||||||||||||||||
Pronunciation | /ˈlɪθiəm/, LI-thee-əm | ||||||||||||||||||||||||
Element category | alkali metal | ||||||||||||||||||||||||
Group, period, block | 1, 2, s | ||||||||||||||||||||||||
Standard atomic weight | 6.941(2) g·mol−1 | ||||||||||||||||||||||||
Electron configuration | 1s2 2s1 | ||||||||||||||||||||||||
Electrons per shell | 2, 1 (Image) | ||||||||||||||||||||||||
Physical properties | |||||||||||||||||||||||||
Phase | solid | ||||||||||||||||||||||||
Density (near r.t.) | 0.534 g·cm−3 | ||||||||||||||||||||||||
Liquid density at m.p. | 0.512 g·cm−3 | ||||||||||||||||||||||||
Melting point | 453.69 K, 180.54 °C, 356.97 °F | ||||||||||||||||||||||||
Boiling point | 1615 K, 1342 °C, 2448 °F | ||||||||||||||||||||||||
Critical point | (extrapolated) 3223 K, 67 MPa | ||||||||||||||||||||||||
Heat of fusion | 3.00 kJ·mol−1 | ||||||||||||||||||||||||
Heat of vaporization | 147.1 kJ·mol−1 | ||||||||||||||||||||||||
Specific heat capacity | (25 °C) 24.860 J·mol−1·K−1 | ||||||||||||||||||||||||
Vapor pressure | |||||||||||||||||||||||||
| |||||||||||||||||||||||||
Atomic properties | |||||||||||||||||||||||||
Oxidation states | +1, -1 (strongly basic oxide) | ||||||||||||||||||||||||
Electronegativity | 0.98 (Pauling scale) | ||||||||||||||||||||||||
Ionization energies | 1st: 520.2 kJ·mol−1 | ||||||||||||||||||||||||
2nd: 7298.1 kJ·mol−1 | |||||||||||||||||||||||||
3rd: 11815.0 kJ·mol−1 | |||||||||||||||||||||||||
Atomic radius | 152 pm | ||||||||||||||||||||||||
Covalent radius | 128±7 pm | ||||||||||||||||||||||||
Van der Waals radius | 182 pm | ||||||||||||||||||||||||
Miscellanea | |||||||||||||||||||||||||
Crystal structure | body-centered cubic | ||||||||||||||||||||||||
Magnetic ordering | paramagnetic | ||||||||||||||||||||||||
Electrical resistivity | (20 °C) 92.8 nΩ·m | ||||||||||||||||||||||||
Thermal conductivity | (300 K) 84.8 W·m−1·K−1 | ||||||||||||||||||||||||
Thermal expansion | (25 °C) 46 µm·m−1·K−1 | ||||||||||||||||||||||||
Speed of sound (thin rod) | (20 °C) 6000 m/s | ||||||||||||||||||||||||
Young's modulus | 4.9 GPa | ||||||||||||||||||||||||
Shear modulus | 4.2 GPa | ||||||||||||||||||||||||
Bulk modulus | 11 GPa | ||||||||||||||||||||||||
Mohs hardness | 0.6 | ||||||||||||||||||||||||
CAS registry number | 7439-93-2 | ||||||||||||||||||||||||
Most stable isotopes | |||||||||||||||||||||||||
Main article: Isotopes of lithium | |||||||||||||||||||||||||
|
Characteristics
Physical
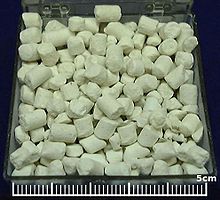

Like the other alkali metals, lithium has a single valence electron that is easily given up to form a cation.[7] Because of this, it is a good conductor of both heat and electricity and highly reactive, though it is the least reactive of the alkali metals due to the proximity of its valence electron to its nucleus.[7]
Lithium is soft enough to be cut with a knife, and it is the lightest of the metals of the periodic table. When cut, it possesses a silvery-white color that quickly changes to gray due to oxidation.[7] It also has a low density (approximately 0.534 g/cm3) and thus will float on water, with which it reacts easily. This reaction is energetic, forming hydrogen gas and lithium hydroxide in aqueous solution.[7] Because of its reactivity with water, lithium is usually stored under cover of a dense hydrocarbon, often petroleum jelly; though the heavier alkaline metals can be stored in less dense substances, such as mineral oil, lithium is not dense enough to be fully submerged in these liquids.[8]
Lithium possesses a low coefficient of thermal expansion and the highest specific heat capacity of any solid element. Lithium is superconductive below 400 μK at standard pressure[9] and at higher temperatures (more than 9 kelvins) at very high pressures (over 200,000 atmospheres)[10] At cryogenic temperatures, lithium, like sodium, undergoes diffusionless phase change transformations. At 4.2K it has a rhombohedral crystal system (with a nine-layer repeat spacing)[11]; at higher temperatures it transforms to face-centered cubic and then body-centered cubic. At liquid-helium temperatures (4 K) the rhombohedral structure is the most prevalent.
Chemical
In moist air, lithium metal rapidly tarnishes to form a black coating of lithium hydroxide (LiOH and LiOH·H2O), lithium nitride (Li3N) and lithium carbonate (Li2CO3, the result of a secondary reaction between LiOH and CO2).[12]
When placed over a flame, lithium gives off a striking crimson color, but when it burns strongly the flame becomes a brilliant silver. Lithium will ignite and burn in oxygen when exposed to water or water vapours.[13]
Lithium metal is flammable, and it is potentially explosive when exposed to air and especially to water, though less so than the other alkali metals. The lithium-water reaction at normal temperatures is brisk but not violent, though the hydrogen produced can ignite. As with all alkali metals, lithium fires are difficult to extinguish, requiring dry powder fire extinguishers, specifically Class D type (see Types of extinguishing agents). Lithium is the only metal which reacts with nitrogen under normal conditions.
[edit] Lithium compounds
![]() | This section requires expansion. |
Lithium has a diagonal relationship with magnesium, an element of similar atomic and ionic radius. Chemical resemblances between the two metals include the formation of a nitride by reaction with N2, the formation of an oxide when burnt in O2, salts with similar solubilities, and thermal instability of the carbonates and nitrides.[12]
Isotopes
Naturally occurring lithium is composed of two stable isotopes, 6Li and 7Li, the latter being the more abundant (92.5% natural abundance).[7][8][14] Both natural isotopes have anomalously low nuclear binding energy per nucleon compared to the next lighter and heavier elements, helium and beryllium, which means that alone among stable light elements, lithium can produce net energy through nuclear fission. Seven radioisotopes have been characterized, the most stable being 8Li with a half-life of 838 ms and 9Li with a half-life of 178.3 ms. All of the remaining radioactive isotopes have half-lives that are shorter than 8.6 ms. The shortest-lived isotope of lithium is 4Li, which decays through proton emission and has a half-life of 7.58043 × 10−23 s.
7Li is one of the primordial elements (or, more properly, primordial isotopes) produced in Big Bang nucleosynthesis. A small amount of both 6Li and 7Li are produced in stars, but are thought to be burned as fast as it is produced.[15] Additional small amounts of lithium of both 6Li and 7Li may be generated from solar wind, cosmic rays, and early solar system 7Be and 10Be radioactive decay.[16] 7Li can also be generated in carbon stars.[17]
Lithium isotopes fractionate substantially during a wide variety of natural processes,[18] including mineral formation (chemical precipitation), metabolism, and ion exchange. Lithium ions substitute for magnesium and iron in octahedral sites in clay minerals, where 6Li is preferred to 7Li, resulting in enrichment of the light isotope in processes of hyperfiltration and rock alteration. The exotic 11Li is known to exhibit a nuclear halo.
History and etymology
Petalite (LiAlSi4O10, which is lithium aluminium silicate) was first discovered in 1800 by the Brazilian chemist José Bonifácio de Andrade e Silva, who discovered this mineral in a mine on the island of Utö, Sweden.[19][20][21] However, it was not until 1817 that Johan August Arfwedson, then working in the laboratory of the chemist Jöns Jakob Berzelius, detected the presence of a new element while analyzing petalite ore.[22][23][24] This element formed compounds similar to those of sodium and potassium, though its carbonate and hydroxide were less soluble in water and more alkaline.[25] Berzelius gave the alkaline material the name "lithos", from the Greek word λιθoς (transliterated as lithos, meaning "stone"), to reflect its discovery in a solid mineral, as opposed to sodium and potassium, which had been discovered in plant tissues. The name of this element was later standardized as "lithium".[7][20][24] Arfwedson later showed that this same element was present in the minerals spodumene and lepidolite.[20] In 1818, Christian Gmelin was the first man to observe that lithium salts give a bright red color in flame.[20] However, both Arfwedson and Gmelin tried and failed to isolate the element from its salts.[20][24][26] This element, lithium, was not isolated until 1821, when William Thomas Brande isolated the element by performing electrolysis on lithium oxide, a process that had previously been employed by the chemist Sir Humphry Davy to isolate the alkali metals potassium and sodium.[8][26][27] Brande also described some pure salts of lithium, such as the chloride, and he performed an estimate of its atomic weight. In 1855, larger quantities of lithium were produced through the electrolysis of lithium chloride by Robert Bunsen and Augustus Matthiessen.[20] The discovery of this procedure henceforth led to commercial production of lithium metal, beginning in 1923 by the German company Metallgesellschaft AG, which performed an electrolysis of a liquid mixture of lithium chloride and potassium chloride.[20][28]
The production and use of lithium underwent several drastic changes in history. The first major application of lithium became high temperature grease for aircraft engines or similar applications in World War II and shortly after. This small market was supported by several small mining operations mostly in the United States. The demand for lithium increased dramatically when in the beginning of the cold war the need for the production of nuclear fusion weapons arose and the dominant fusion material tritium had to be made by irradiating lithium-6. The United States became the prime producer of lithium in the period between the late 1950s and the mid 1980s. At the end the stockpile of lithium was roughly 42,000 tons of lithium hydroxide. The stockpiled lithium was depleted in lithium-6 by 75% .[29]
Lithium was used to decrease the melting temperature of glass and to improve the melting behavior of aluminium oxide when using the Hall-Héroult process.[30][30] These two uses dominated the market until the middle of the 1990s. After the end of the nuclear arms race the demand for lithium decreased and the sale of Department of Energy stockpiles on the open market further reduced prices.[29] Then, in the mid 1990's several companies started to extract lithium from brine; this method proved to be less expensive than underground or even open pit mining. Most of the mines closed or shifted their focus to other materials as only the ore from zoned pegmatites could be mined for a competitive price. For example, the US mines near Kings Mountain, North Carolina closed before the turn of the century. The use in lithium ion batteries increased the demand for lithium and became the dominant use in 2007.[29] New companies have expanded brine extraction efforts to meet the rising demand.[31]
Occurrence
![]() ![]() | ||||||||||||||||||||||||||||||||||||||||||||||||
|
Astronomical occurrence
According to modern cosmological theory, both stable isotopes of lithium—6Li and 7Li—were among the 3 elements synthesized in the Big Bang. Though the amount of lithium generated in Big Bang nucleosynthesis is dependent upon the number of photons per baryon, for accepted values the lithium abundance can be calculated, and there is a "cosmological lithium discrepancy" in the universe: older stars seem to have less lithium than they should, and some younger stars have far more. The lack of lithium in older stars is apparently caused by the "mixing" of lithium into the interior of stars, where it is destroyed.[1] Furthermore, lithium is produced in younger stars. Though it transmutes into two atoms of helium due to collision with a proton at temperatures above 2.4 million degrees Celsius (most stars easily attain this temperature in their interiors), lithium is more abundant than predicted in later-generation stars, for causes not yet completely understood.[8]
Though it was one of the 3 first elements to be synthesized in the Big Bang, lithium, as well as beryllium and boron are markedly less abundant than the elements with either lower or higher atomic number. This is due to the low temperature necessary to destroy lithium, and a lack of common processes to produce it.[33]
Lithium is also found in brown dwarf stars and certain anomalous orange stars. Because lithium is present in cooler, less-massive brown dwarf stars, but is destroyed in hotter red dwarf stars, its presence in the stars' spectra can be used in the "lithium test" to differentiate the two, as both are smaller than the Sun.[8][34][35] Certain orange stars can also contain a high concentration of lithium. Those orange stars found to have a higher than usual concentration of lithium (such as Centaurus X-4) orbit massive objects—neutron stars or black holes—whose gravity evidently pulls heavier lithium to the surface of a hydrogen-helium star, causing more lithium to be observed.[8]
Occurrence on Earth
Lithium is widely distributed on Earth but does not naturally occur in elemental form due to its high reactivity.[7] Estimates for crustal content range from 20 to 70 ppm by weight.[12] In keeping with its name, lithium forms a minor part of igneous rocks, with the largest concentrations in granites. Granitic pegmatites also provide the greatest abundance of lithium-containing minerals, with spodumene and petalite being the most commercially viable sources.[12] A newer source for lithium is hectorite clay, the only active development of which is through the Western Lithium Corporation in the United States.[36]
According to the Handbook of Lithium and Natural Calcium, "Lithium is a comparatively rare element, although it is found in many rocks and some brines, but always in very low concentrations. There are a fairly large number of both lithium mineral and brine deposits but only comparatively a few of them are of actual or potential commercial value. Many are very small, others are too low in grade."[37] At 20 mg lithium per kg of Earth's crust [38], lithium is the 25th most abundant element. Nickel and lead have the about the same abundance.
The largest reserve base of lithium is in the Salar de Uyuni area of Bolivia, which has 5.4 million tons. According to the US Geological Survey, the production and reserves of lithium in metric tons are as follows[32][39]:
Contrary to the USGS data in the table, other estimates put Chile's reserve base at 7,520,000 metric tons of lithium, and Argentina's at 6,000,000 metric tons.[40]
Seawater contains an estimated 230 billion tons of lithium, though at a low concentration of 0.1 to 0.2 ppm.[41]
Production



Since the end of World War II lithium metal production has greatly increased. The metal is separated from other elements in igneous minerals such as those above. Lithium salts are extracted from the water of mineral springs, brine pools and brine deposits.
The metal is produced electrolytically from a mixture of fused lithium chloride and potassium chloride. In 1998 it was about 95 US$ / kg (or 43 US$/pound).[42]
Deposits of lithium are found in South America throughout the Andes mountain chain. Chile is the leading lithium metal producer, followed by Argentina. Both countries recover the lithium from brine pools. In the United States lithium is recovered from brine pools in Nevada.[43] Nearly half the world's known reserves are located in Bolivia, a nation sitting along the central eastern slope of the Andes. In 2009 Bolivia is negotiating with Japanese, French, and even Korean firms to begin extraction.[44] According to the US Geological Survey, Bolivia's Uyuni Desert has 5.4 million tons of lithium, which can be used to make batteries for hybrid and electric vehicles.[44][45]
China may emerge as a significant producer of brine-source lithium carbonate around 2010. There is potential production of up to 55,000 tons per year if projects in Qinghai province and Tibet proceed.[46]
The total amount of lithium recoverable from global reserves has been estimated at 35 million tonnes, which includes 15 million tons of the known global lithium reserve base.[47]
In 1976 a National Research Council Panel estimated lithium resources at 10.6 million tons for the Western World.[48] With the inclusion of Russian and Chinese resources as well as new discoveries in Australia, Serbia, Argentina and the United States, the total had nearly tripled by 2008.[49][50]
Applications
Because of its specific heat capacity, the highest of all solids, lithium is often used in coolants for heat transfer applications.
In the latter years of the 20th century lithium became important as an anode material. Used in lithium-ion batteries because of its high electrochemical potential, a typical cell can generate approximately 3 volts, compared with 2.1 volts for lead/acid or 1.5 volts for zinc-carbon cells. Because of its low atomic mass, it also has a high charge- and power-to-weight ratio.
Lithium is also used in the pharmaceutical and fine-chemical industry in the manufacture of organolithium reagents, which are used both as strong bases and as reagents for the formation of carbon-carbon bonds. Organolithiums are also used in polymer synthesis as catalysts/initiators[51] in anionic polymerization of unfunctionalised olefins.[52][53][54]
Lithium-6 is valued as a source material for tritium production and as a neutron absorber in nuclear fusion. Natural lithium contains about 7.5 percent lithium-6. Large amounts of lithium-6 have been produced by isotope separation for use in nuclear weapons. Lithium-7 gained interest for use in nuclear reactor coolants.
Medical use
Lithium salts were used during the 19th century to treat gout. Lithium salts such as lithium carbonate (Li2CO3), lithium citrate, and lithium orotate are mood stabilizers. They are used in the treatment of bipolar disorder since, unlike most other mood altering drugs, they counteract both mania and depression. Lithium can also be used to augment antidepressants. Because of Lithium's nephrogenic diabetes insipidus effects, it can be used to help treat the syndrome of inappropriate antidiuretic hormone hypersecretion (SIADH). It was also sometimes prescribed as a preventive treatment for migraine disease and cluster headaches.[55]
The active principle in these salts is the lithium ion Li+. Although this ion has a smaller diameter than either Na+ or K+, in a watery environment like the cytoplasmic fluid, Li+ binds to the oxygen atoms of water, making it effectively larger than either Na+ or K+ ions. How Li+ works in the central nervous system is still a matter of debate. Li+ elevates brain levels of tryptophan, 5-HT (serotonin), and 5-HIAA (a serotonin metabolite). Serotonin is related to mood stability. Li+ also reduces catecholamine activity in the brain (associated with brain activation and mania), by enhancing reuptake and reducing release. Therapeutically useful amounts of lithium (~ 0.6 to 1.2 mmol/l) are only slightly lower than toxic amounts (>1.5 mmol/l), so the blood levels of lithium must be carefully monitored during treatment to avoid toxicity.
Common side effects of lithium treatment include muscle tremors, twitching, ataxia[56] and hypothyroidism. Long term use is linked to hyperparathyroidism[57], hypercalcemia (bone loss), hypertension, damage of tubuli in the kidney, nephrogenic diabetes insipidus (polyuria and polydipsia) and/or glomerular damage - even to the point of uremia[58], seizures[59] and weight gain.[60] Some of the side-effects are a result of the increased elimination of potassium.
There appears to be an increased risk of Ebstein (cardiac) Anomaly in infants born to women taking lithium during the first trimester of pregnancy.
According to a study in 2009 at Oita University in Japan and published in the British Journal of Psychiatry, communities whose water contained larger amounts of lithium had significantly lower suicide rates[61][62][63][64] but did not address whether lithium in drinking water causes the negative side effects associated with higher doses of the element.[65]
Other uses


- Electrical and electronic uses:
- Lithium batteries are disposable (primary) batteries with lithium metal or lithium compounds as an anode. Lithium batteries are not to be confused with lithium-ion batteries, which are high energy-density rechargeable batteries. Other rechargeable batteries include the lithium-ion polymer battery, lithium iron phosphate battery, and the nanowire battery. New technologies are constantly being announced.
- Lithium niobate is used extensively in telecommunication products such as mobile phones and optical modulators, for such components as resonant crystals. Lithium applications are used in more than 60% of mobile phones.[66]
- Chemical uses:
- Lithium chloride and lithium bromide are extremely hygroscopic and are used as desiccants.
- Lithium metal is used in the preparation of organolithium compounds.
- General engineering:
- Lithium stearate is a common all-purpose, high-temperature lubricant.
- When used as a flux for welding or soldering, lithium promotes the fusing of metals during and eliminates the forming of oxides by absorbing impurities. Its fusing quality is also important as a flux for producing ceramics, enamels and glass.
- Alloys of the metal with aluminium, cadmium, copper and manganese are used to make high-performance aircraft parts (see also Lithium-aluminium alloys).
- Optics:
- Lithium is sometimes used in focal lenses, including spectacles and the glass for the 200-inch (5.08 m) telescope at Mt. Palomar.[citation needed]
- The high non-linearity of lithium niobate also makes it useful in non-linear optics applications.
- Lithium fluoride, artificially grown as crystal, is clear and transparent and often used in specialist optics for IR, UV and VUV (vacuum UV) applications. It has the lowest refractive index and the farthest transmission range in the deep UV of all common materials.
- Rocketry:
- Metallic lithium and its complex hydrides, such a Li[AlH4], are used as high energy additives to rocket propellants[3].
- Lithium peroxide, lithium nitrate, lithium chlorate and lithium perchlorate are used as oxidizers in rocket propellants, and also in oxygen candles that supply submarines and space capsules with oxygen.[67]
- Nuclear applications:
- Lithium deuteride was the fusion fuel of choice in early versions of the hydrogen bomb. When bombarded by neutrons, both 6Li and 7Li produce tritium—this reaction, which was not fully understood when hydrogen bombs were first tested, was responsible for the runaway yield of the Castle Bravo nuclear test. Tritium fuses with deuterium in a fusion reaction that is relatively easy to achieve. Although details remain secret, lithium-6 deuteride still apparently plays a role in modern nuclear weapons, as a fusion material.
- Lithium fluoride (highly enriched in the common isotope lithium-7) forms the basic constituent of the preferred fluoride salt mixture (LiF-BeF2) used in liquid-fluoride nuclear reactors. Lithium fluoride is exceptionally chemically stable and LiF/BeF2 mixtures have low melting points and the best neutronic properties of fluoride salt combinations appropriate for reactor use.[clarification needed] Finely divided lithium fluoride powder has been used for thermoluminescent radiation dosimetry (TLD): When a sample of such is exposed to radiation, it accumulates crystal defects which, when heated, resolve via a release of bluish light whose intensity is proportional to the absorbed dose, thus allowing this to be quantified (see Eugene Tochilin, et al.).
- In conceptualized nuclear fusion power plants, lithium will be used to produce tritium in magnetically confined reactors using deuterium and tritium as the fuel. Tritium does not occur naturally and will be produced by surrounding the reacting plasma with a 'blanket' containing lithium where neutrons from the deuterium-tritium reaction in the plasma will react with the lithium to produce more tritium. 6Li + n → 4He + 3H. Various means of doing this will be tested at the ITER reactor being built at Cadarache, France.
- Lithium is used as a source for alpha particles, or helium nuclei. When 7Li is bombarded by accelerated protons 8Be is formed, which undergoes spontaneous fission to form two alpha particles. This was the first man-made nuclear reaction, produced by Cockroft and Walton in 1929.
- Other uses:
- Lithium hydroxide (LiOH) is an important compound of lithium obtained from lithium carbonate (Li2CO3). It is a strong base, and when heated with a fat it produces a lithium soap. Lithium soap has the ability to thicken oils, and it is used to manufacture lubricating greases.
- Lithium hydroxide and lithium peroxide are used in confined areas, such as aboard spacecraft and submarines, for air purification. Lithium hydroxide absorbs carbon dioxide from the air by reacting with it to form lithium carbonate, and is preferred over other alkaline hydroxides for its low weight. Lithium peroxide (Li2O2) in presence of moisture not only absorbs carbon dioxide to form lithium carbonate, but also releases oxygen. For example 2 Li2O2 + 2 CO2 → 2 Li2CO3 + O2.
- Lithium compounds are used as pyrotechnic colorants and oxidizers in red fireworks and flares.
- The Mark 50 Torpedo Stored Chemical Energy Propulsion System (SCEPS) uses a small tank of sulfur hexafluoride gas which is sprayed over a block of solid lithium. The reaction generates enormous heat which is used to generate steam from seawater. The steam propels the torpedo in a closed Rankine cycle.[68]
Precautions

Lithium metal is corrosive and requires special handling to avoid skin contact. Breathing lithium dust or lithium compounds (which are often alkaline) initially irritate the nose and throat, while higher exposure can cause a buildup of fluid in the lungs, leading to pulmonary edema. The metal itself is a handling hazard because of the caustic hydroxide produced when it is in contact with moisture. Lithium is safely stored in non-reactive compounds such as naphtha.[69]
NFPA 704 |
---|
![]() 2 3 2 |
Fire diamond for lithium metal |
Regulation
Some jurisdictions limit the sale of lithium batteries, which are the most readily available source of lithium metal for ordinary consumers. Lithium can be used to reduce pseudoephedrine and ephedrine to methamphetamine in the Birch reduction method, which employs solutions of alkali metals dissolved in anhydrous ammonia.
Carriage and shipment of some kinds of lithium batteries may be prohibited aboard certain types of transportation (particularly aircraft) because of the ability of most types of lithium batteries to fully discharge very rapidly when short-circuited, leading to overheating and possible explosion in a process called thermal runaway. Most consumer lithium batteries have thermal overload protection built-in to prevent this type of incident, or their design inherently limits short-circuit currents. Internal shorts have been known to develop due to manufacturing defects or damage to batteries that can lead to spontaneous thermal runaway.[70]